Formation of the Solar System and Extrasolar Planets 1
47 Slides4.19 MB
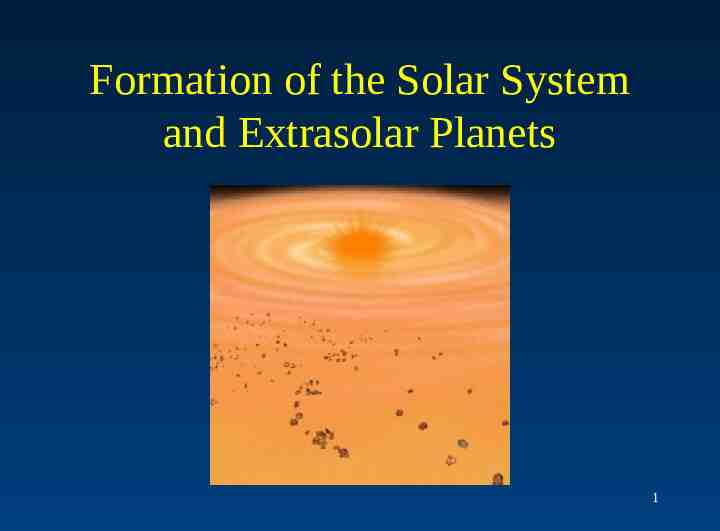
Formation of the Solar System and Extrasolar Planets 1
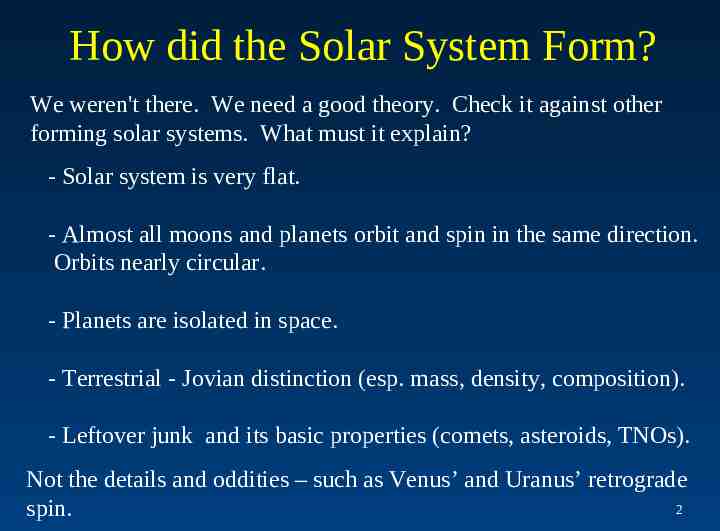
How did the Solar System Form? We weren't there. We need a good theory. Check it against other forming solar systems. What must it explain? - Solar system is very flat. - Almost all moons and planets orbit and spin in the same direction. Orbits nearly circular. - Planets are isolated in space. - Terrestrial - Jovian distinction (esp. mass, density, composition). - Leftover junk and its basic properties (comets, asteroids, TNOs). Not the details and oddities – such as Venus’ and Uranus’ retrograde 2 spin.
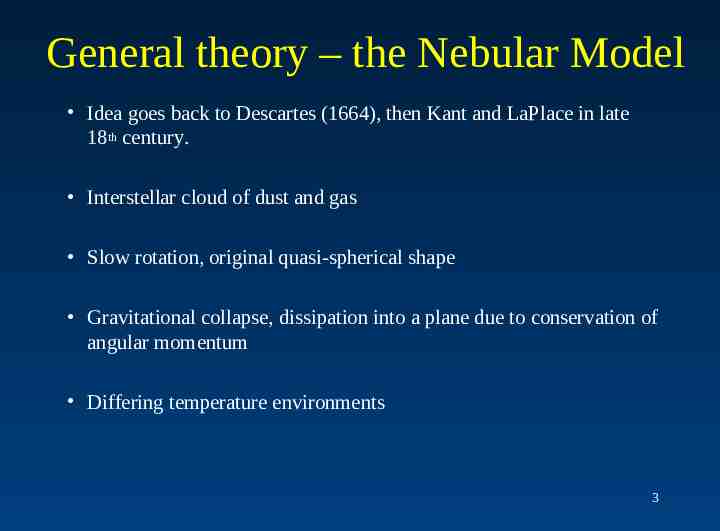
General theory – the Nebular Model Idea goes back to Descartes (1664), then Kant and LaPlace in late 18th century. Interstellar cloud of dust and gas Slow rotation, original quasi-spherical shape Gravitational collapse, dissipation into a plane due to conservation of angular momentum Differing temperature environments 3
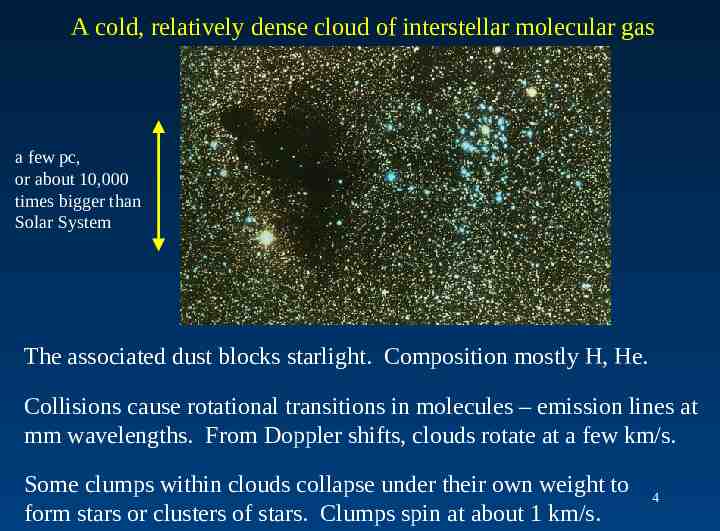
A cold, relatively dense cloud of interstellar molecular gas a few pc, or about 10,000 times bigger than Solar System The associated dust blocks starlight. Composition mostly H, He. Collisions cause rotational transitions in molecules – emission lines at mm wavelengths. From Doppler shifts, clouds rotate at a few km/s. Some clumps within clouds collapse under their own weight to form stars or clusters of stars. Clumps spin at about 1 km/s. 4
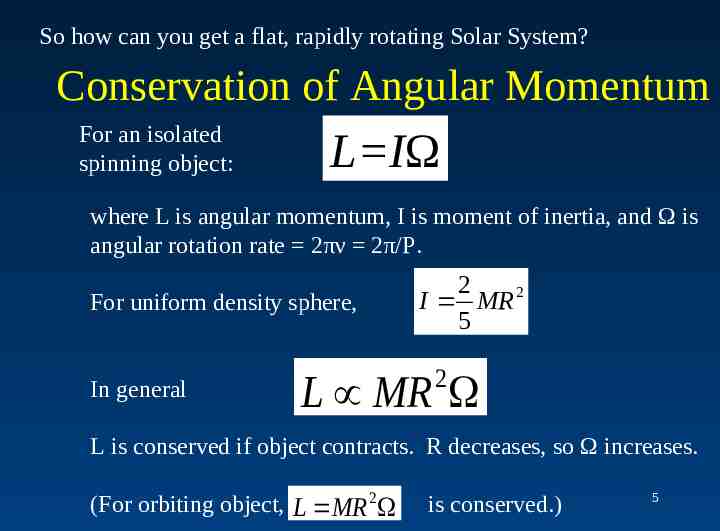
So how can you get a flat, rapidly rotating Solar System? Conservation of Angular Momentum For an isolated spinning object: L IΩ where L is angular momentum, I is moment of inertia, and Ω is angular rotation rate 2πν 2π/P. For uniform density sphere, In general 2 I MR 2 5 2 L MR L is conserved if object contracts. R decreases, so Ω increases. (For orbiting object, L MR 2 is conserved.) 5
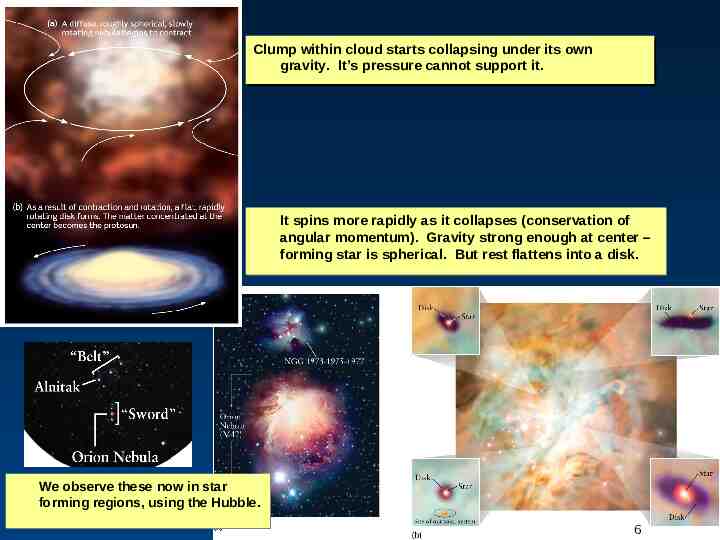
Clump Clump within within cloud cloud starts starts collapsing collapsing under under its its own own gravity. gravity. It’s It’s pressure pressure cannot cannot support support it. it. It spins more rapidly as it collapses (conservation of angular momentum). Gravity strong enough at center – forming star is spherical. But rest flattens into a disk. We observe these now in star forming regions, using the Hubble. 6
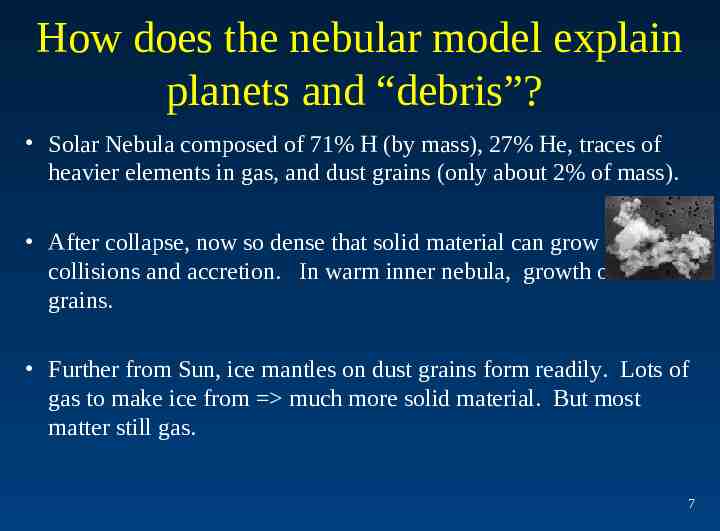
How does the nebular model explain planets and “debris”? Solar Nebula composed of 71% H (by mass), 27% He, traces of heavier elements in gas, and dust grains (only about 2% of mass). After collapse, now so dense that solid material can grow by collisions and accretion. In warm inner nebula, growth of dust grains. Further from Sun, ice mantles on dust grains form readily. Lots of gas to make ice from much more solid material. But most matter still gas. 7
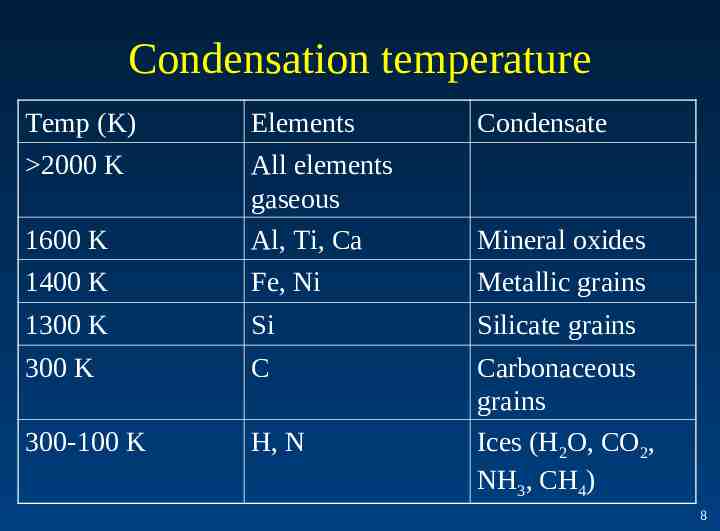
Condensation temperature Temp (K) Elements Condensate 2000 K 1600 K All elements gaseous Al, Ti, Ca Mineral oxides 1400 K Fe, Ni Metallic grains 1300 K Si Silicate grains 300 K C 300-100 K H, N Carbonaceous grains Ices (H2O, CO2, NH3, CH4) 8
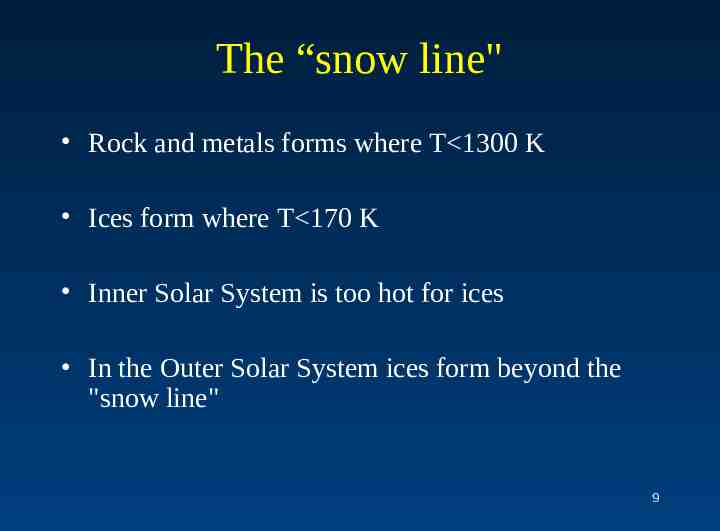
The “snow line" Rock and metals forms where T 1300 K Ices form where T 170 K Inner Solar System is too hot for ices In the Outer Solar System ices form beyond the "snow line" 9
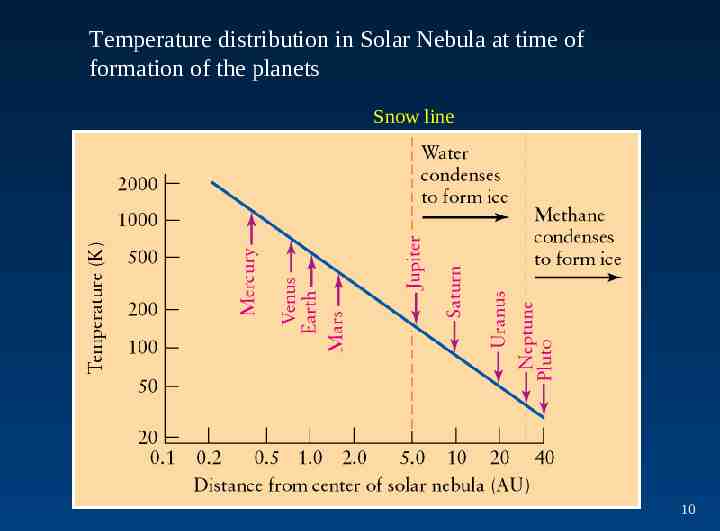
Temperature distribution in Solar Nebula at time of formation of the planets Snow line 10
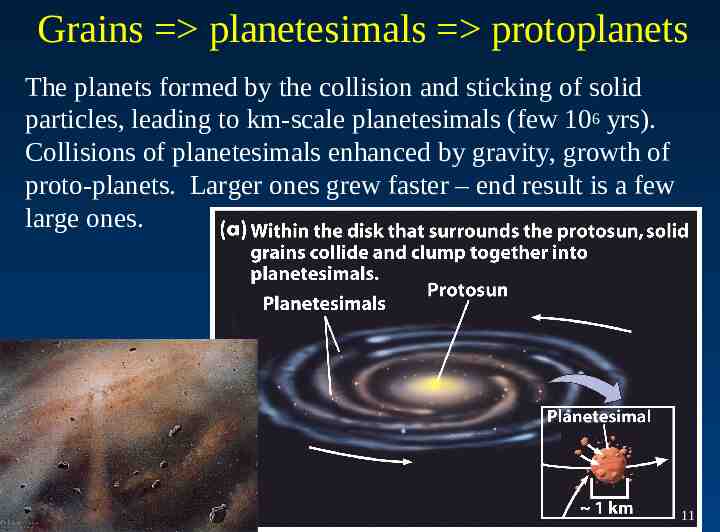
Grains planetesimals protoplanets The planets formed by the collision and sticking of solid particles, leading to km-scale planetesimals (few 106 yrs). Collisions of planetesimals enhanced by gravity, growth of proto-planets. Larger ones grew faster – end result is a few large ones. 11
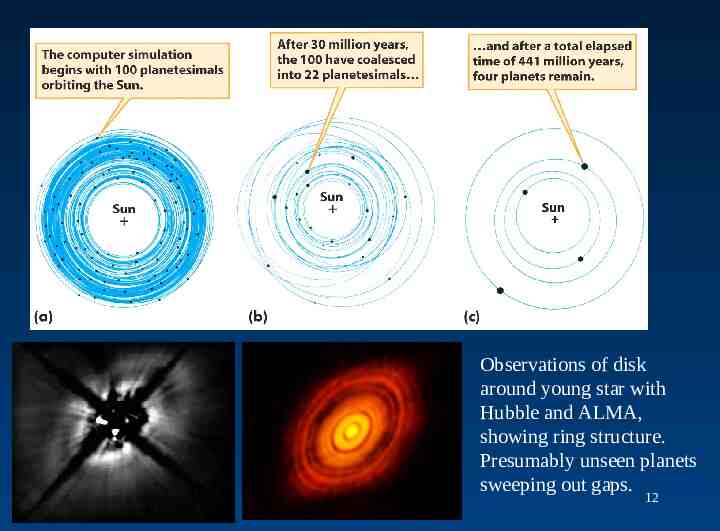
Observations of disk around young star with Hubble and ALMA, showing ring structure. Presumably unseen planets sweeping out gaps. 12
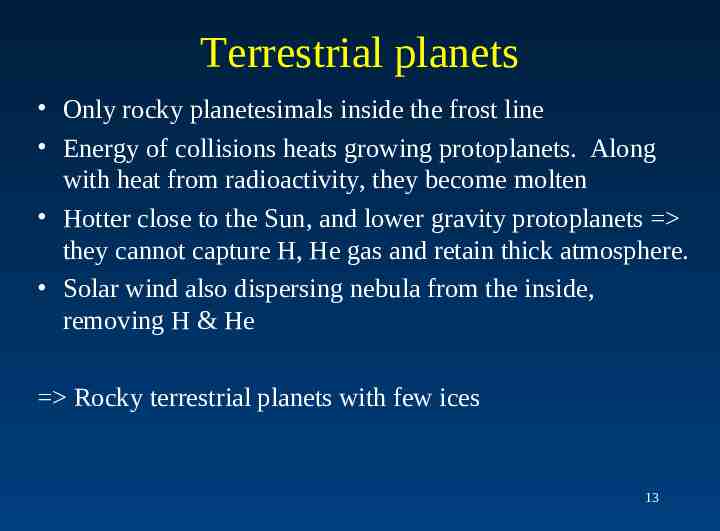
Terrestrial planets Only rocky planetesimals inside the frost line Energy of collisions heats growing protoplanets. Along with heat from radioactivity, they become molten Hotter close to the Sun, and lower gravity protoplanets they cannot capture H, He gas and retain thick atmosphere. Solar wind also dispersing nebula from the inside, removing H & He Rocky terrestrial planets with few ices 13
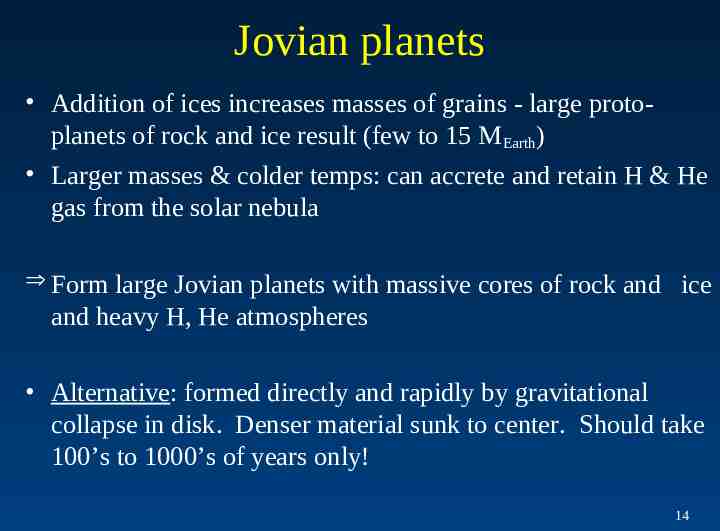
Jovian planets Addition of ices increases masses of grains - large protoplanets of rock and ice result (few to 15 MEarth) Larger masses & colder temps: can accrete and retain H & He gas from the solar nebula Form large Jovian planets with massive cores of rock and ice and heavy H, He atmospheres Alternative: formed directly and rapidly by gravitational collapse in disk. Denser material sunk to center. Should take 100’s to 1000’s of years only! 14
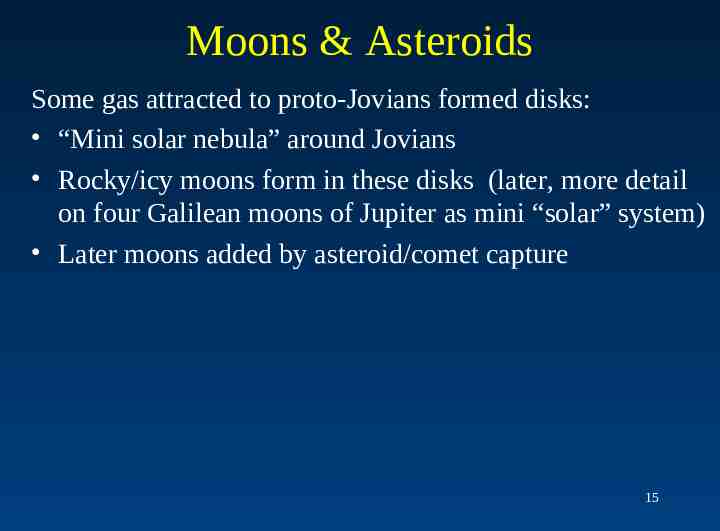
Moons & Asteroids Some gas attracted to proto-Jovians formed disks: “Mini solar nebula” around Jovians Rocky/icy moons form in these disks (later, more detail on four Galilean moons of Jupiter as mini “solar” system) Later moons added by asteroid/comet capture 15
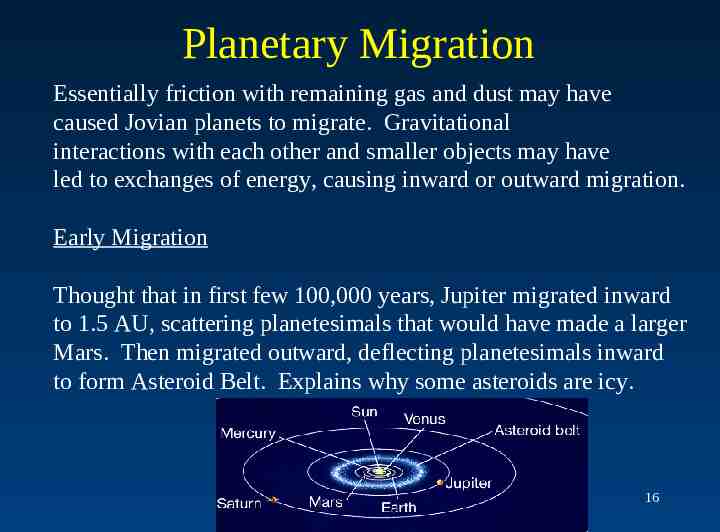
Planetary Migration Essentially friction with remaining gas and dust may have caused Jovian planets to migrate. Gravitational interactions with each other and smaller objects may have led to exchanges of energy, causing inward or outward migration. Early Migration Thought that in first few 100,000 years, Jupiter migrated inward to 1.5 AU, scattering planetesimals that would have made a larger Mars. Then migrated outward, deflecting planetesimals inward to form Asteroid Belt. Explains why some asteroids are icy. 16
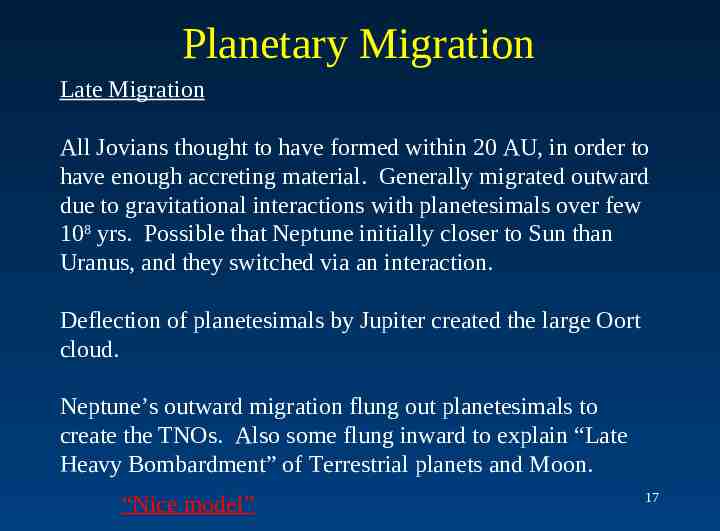
Planetary Migration Late Migration All Jovians thought to have formed within 20 AU, in order to have enough accreting material. Generally migrated outward due to gravitational interactions with planetesimals over few 108 yrs. Possible that Neptune initially closer to Sun than Uranus, and they switched via an interaction. Deflection of planetesimals by Jupiter created the large Oort cloud. Neptune’s outward migration flung out planetesimals to create the TNOs. Also some flung inward to explain “Late Heavy Bombardment” of Terrestrial planets and Moon. “Nice model” 17
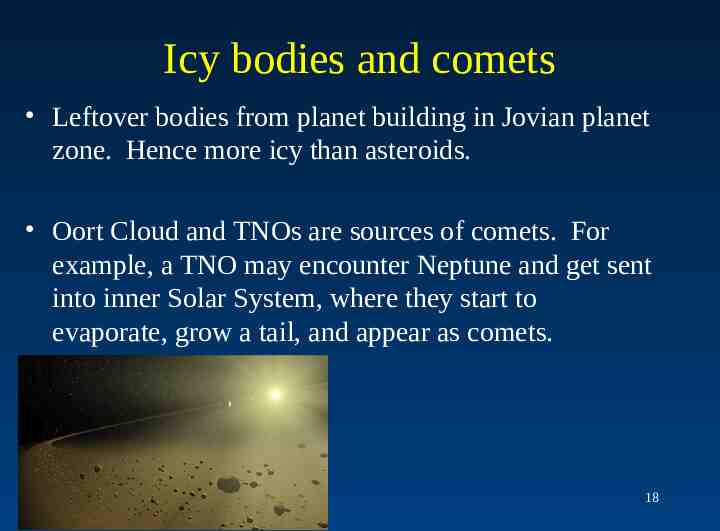
Icy bodies and comets Leftover bodies from planet building in Jovian planet zone. Hence more icy than asteroids. Oort Cloud and TNOs are sources of comets. For example, a TNO may encounter Neptune and get sent into inner Solar System, where they start to evaporate, grow a tail, and appear as comets. 18
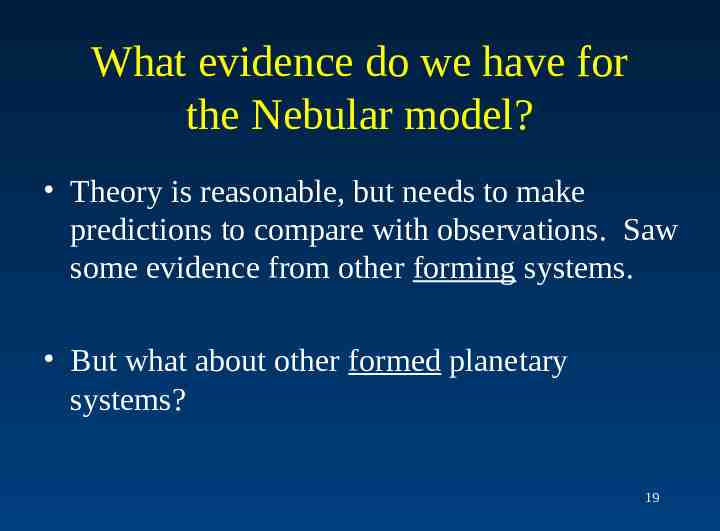
What evidence do we have for the Nebular model? Theory is reasonable, but needs to make predictions to compare with observations. Saw some evidence from other forming systems. But what about other formed planetary systems? 19
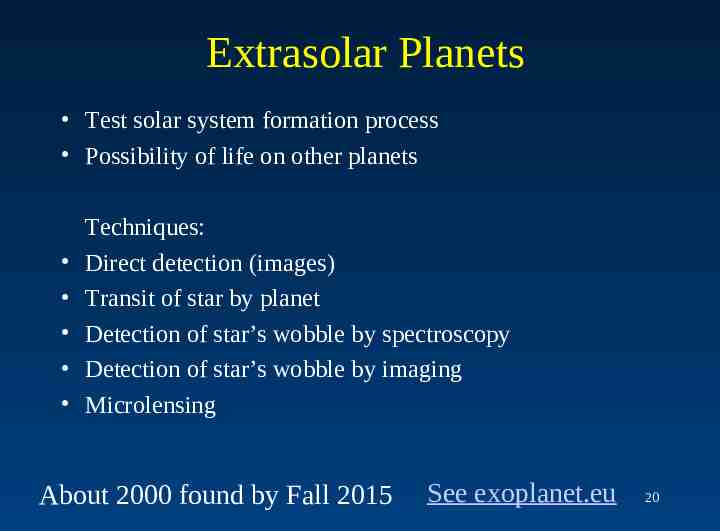
Extrasolar Planets Test solar system formation process Possibility of life on other planets Techniques: Direct detection (images) Transit of star by planet Detection of star’s wobble by spectroscopy Detection of star’s wobble by imaging Microlensing About 2000 found by Fall 2015 See exoplanet.eu 20
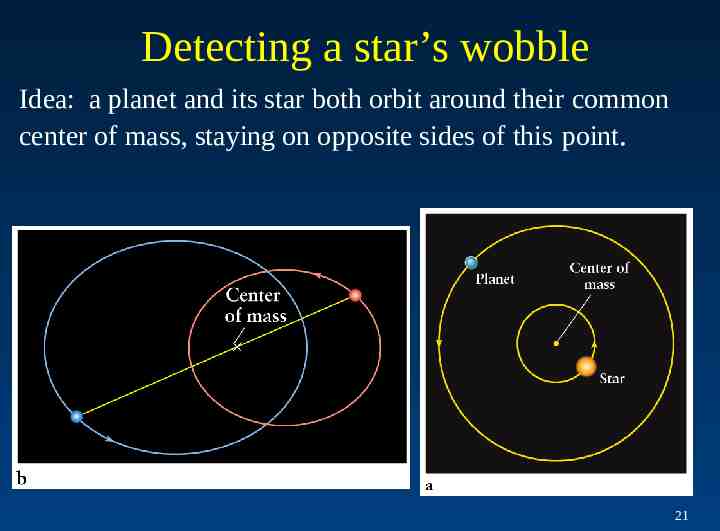
Detecting a star’s wobble Idea: a planet and its star both orbit around their common center of mass, staying on opposite sides of this point. 21
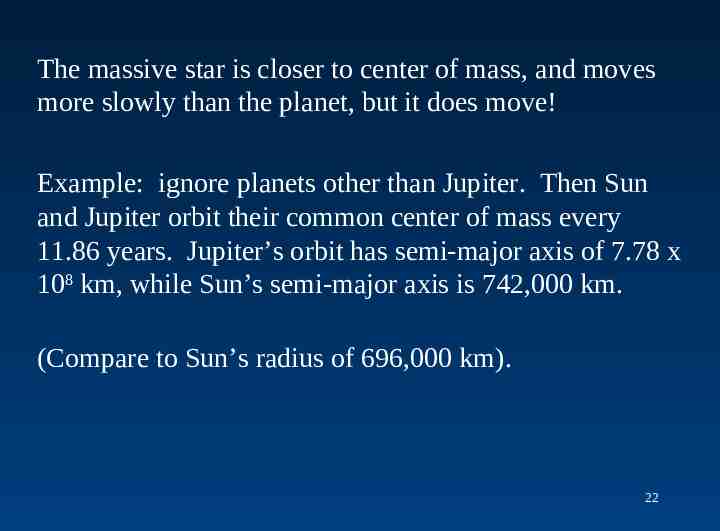
The massive star is closer to center of mass, and moves more slowly than the planet, but it does move! Example: ignore planets other than Jupiter. Then Sun and Jupiter orbit their common center of mass every 11.86 years. Jupiter’s orbit has semi-major axis of 7.78 x 108 km, while Sun’s semi-major axis is 742,000 km. (Compare to Sun’s radius of 696,000 km). 22
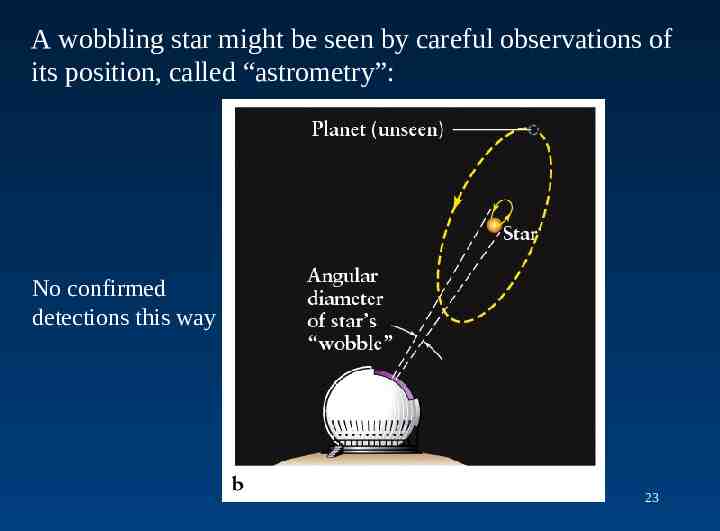
A wobbling star might be seen by careful observations of its position, called “astrometry”: No confirmed detections this way 23
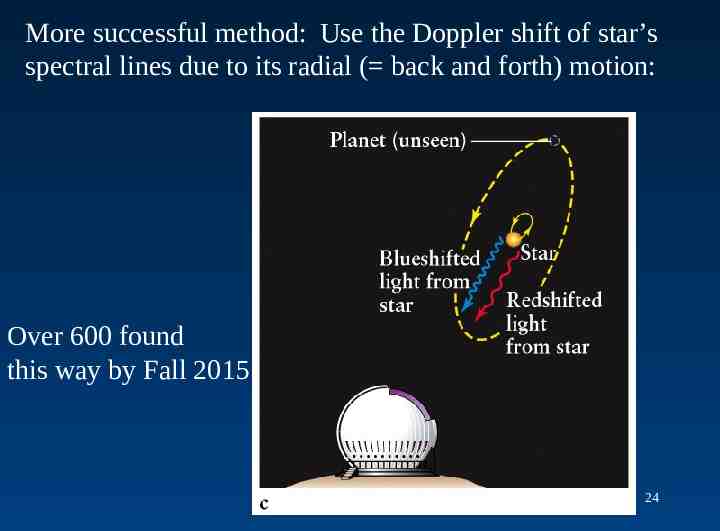
More successful method: Use the Doppler shift of star’s spectral lines due to its radial ( back and forth) motion: Over 600 found this way by Fall 2015 24
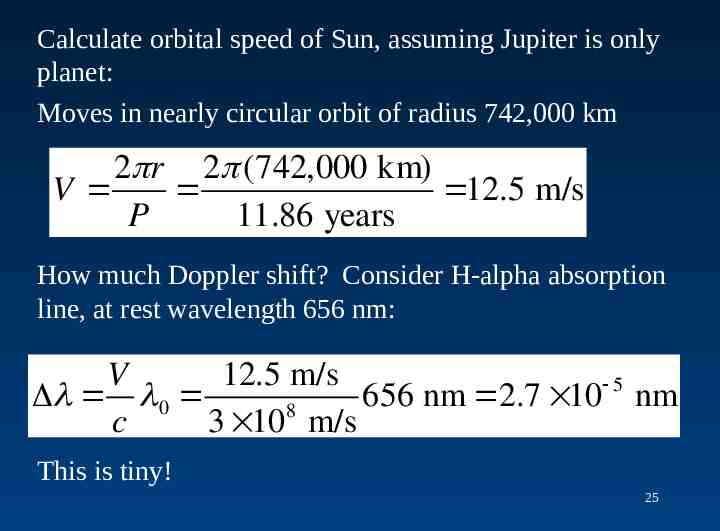
Calculate orbital speed of Sun, assuming Jupiter is only planet: Moves in nearly circular orbit of radius 742,000 km 2 r 2 (742,000 km) V 12.5 m/s P 11.86 years How much Doppler shift? Consider H-alpha absorption line, at rest wavelength 656 nm: V 12.5 m/s 5 0 656 nm 2.7 10 nm 8 c 3 10 m/s This is tiny! 25
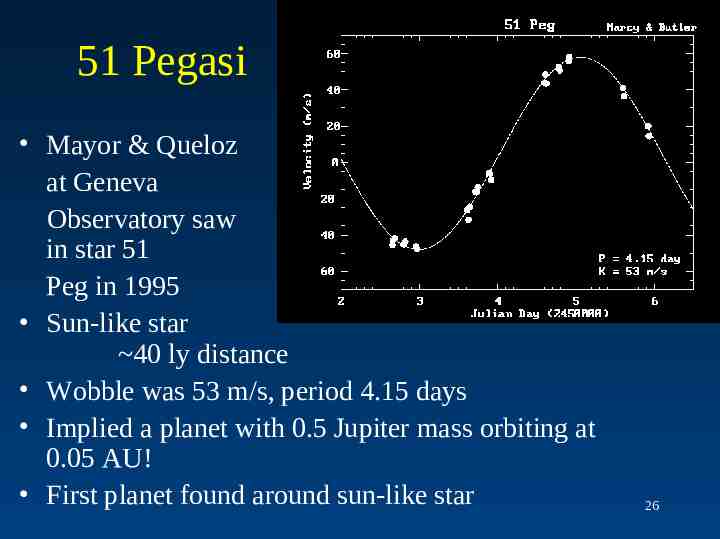
51 Pegasi Mayor & Queloz at Geneva Observatory saw observed wobble in star 51 Peg in 1995 Sun-like star 40 ly distance Wobble was 53 m/s, period 4.15 days Implied a planet with 0.5 Jupiter mass orbiting at 0.05 AU! First planet found around sun-like star 26
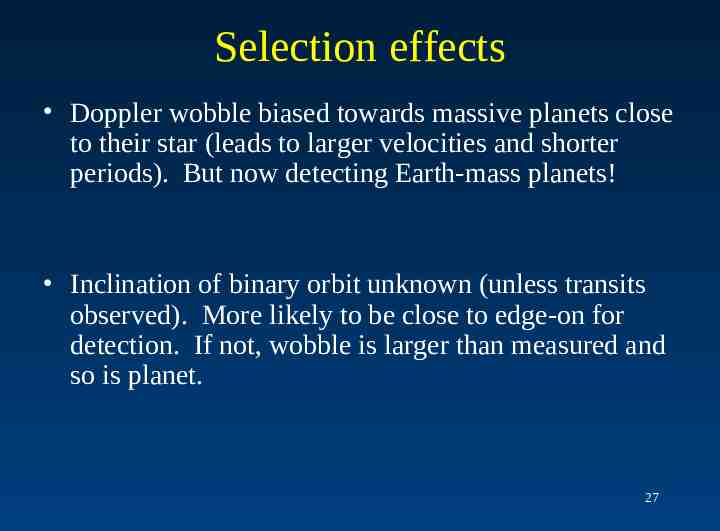
Selection effects Doppler wobble biased towards massive planets close to their star (leads to larger velocities and shorter periods). But now detecting Earth-mass planets! Inclination of binary orbit unknown (unless transits observed). More likely to be close to edge-on for detection. If not, wobble is larger than measured and so is planet. 27
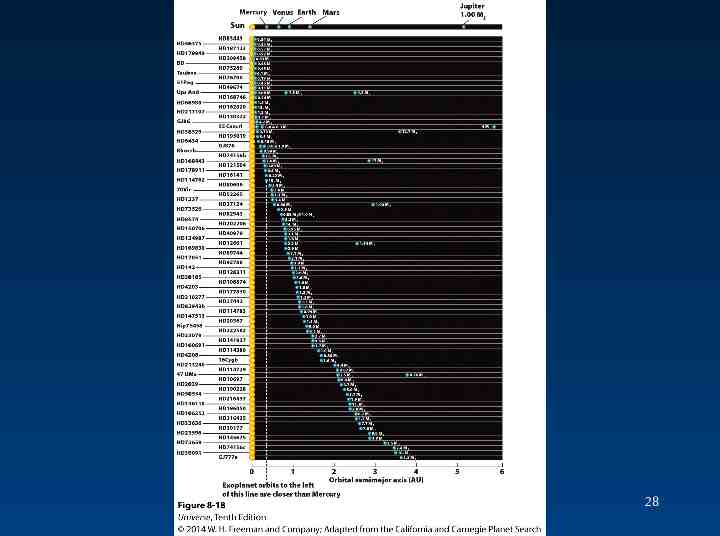
28
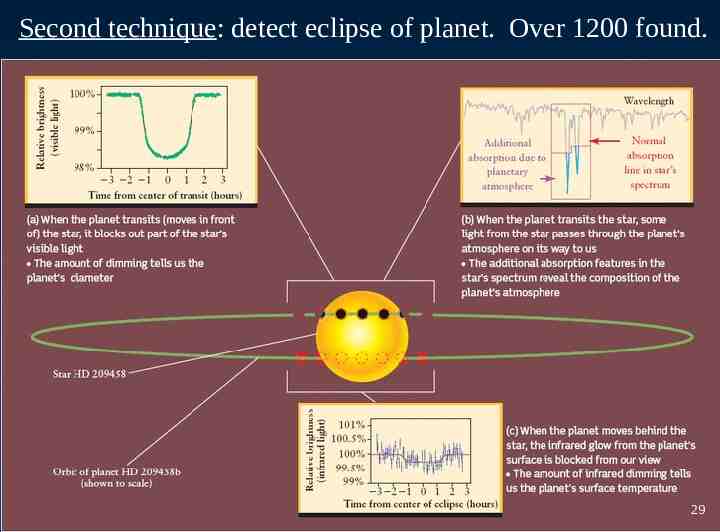
Second technique: detect eclipse of planet. Over 1200 found. 29
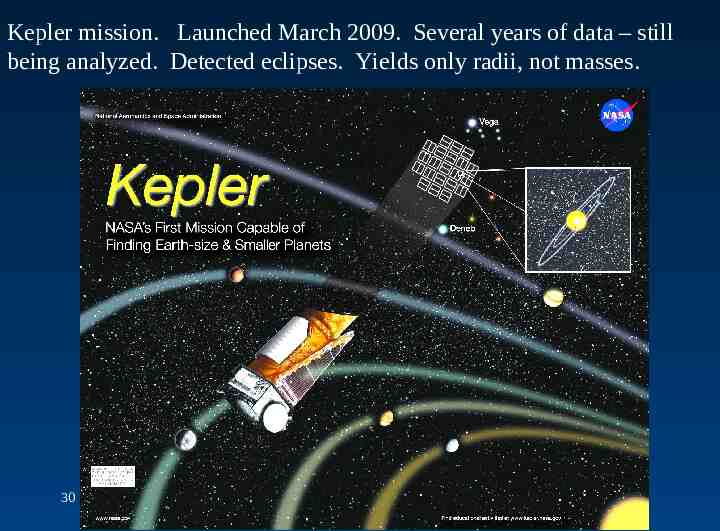
Kepler mission. Launched March 2009. Several years of data – still being analyzed. Detected eclipses. Yields only radii, not masses. 30
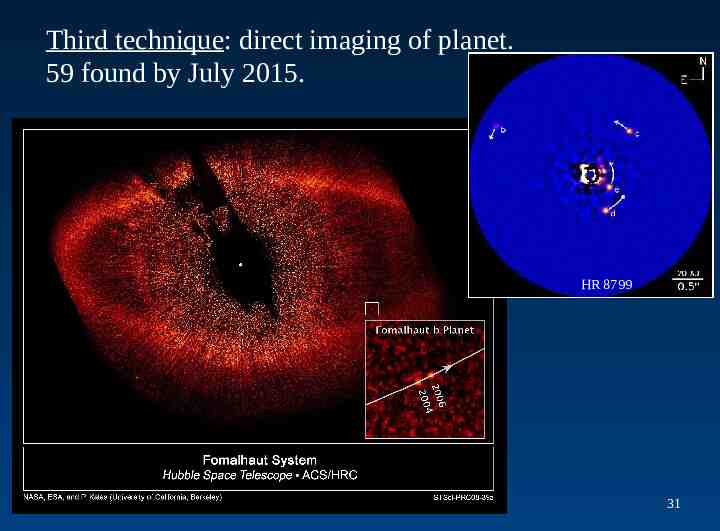
Third technique: direct imaging of planet. 59 found by July 2015. HR 8799 31
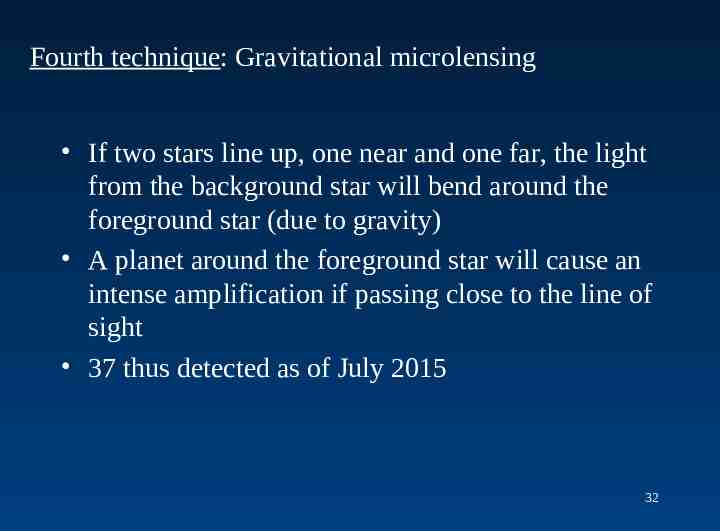
Fourth technique: Gravitational microlensing If two stars line up, one near and one far, the light from the background star will bend around the foreground star (due to gravity) A planet around the foreground star will cause an intense amplification if passing close to the line of sight 37 thus detected as of July 2015 32
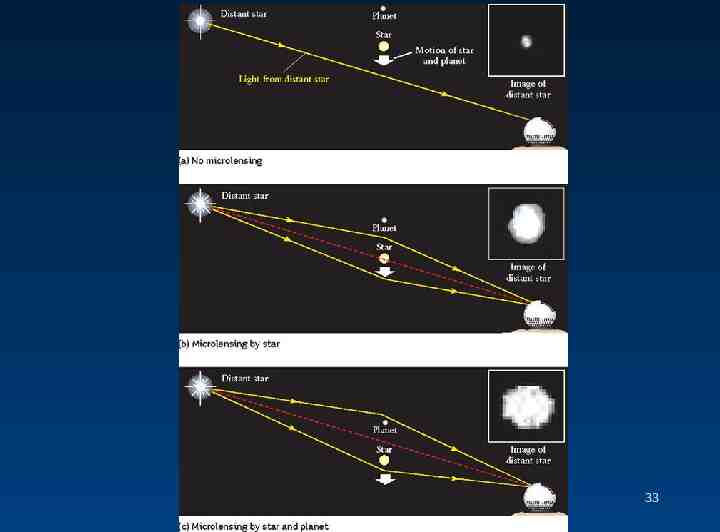
33
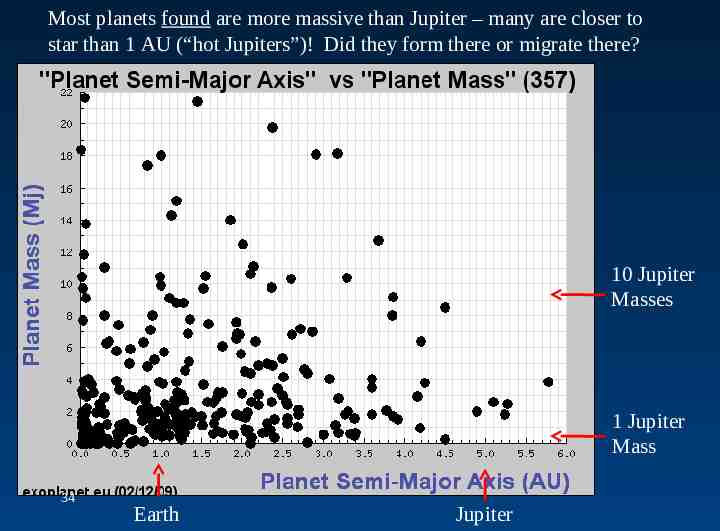
Most planets found are more massive than Jupiter – many are closer to star than 1 AU (“hot Jupiters”)! Did they form there or migrate there? 10 Jupiter Masses 1 Jupiter Mass 34 Earth Jupiter
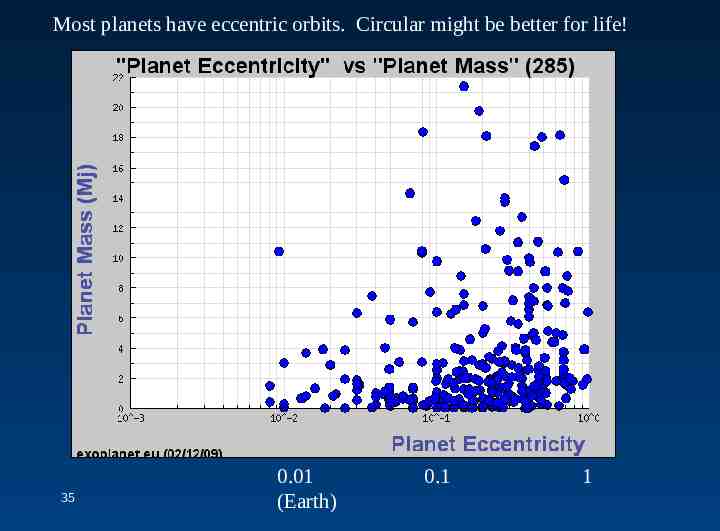
Most planets have eccentric orbits. Circular might be better for life! 35 0.01 (Earth) 0.1 1
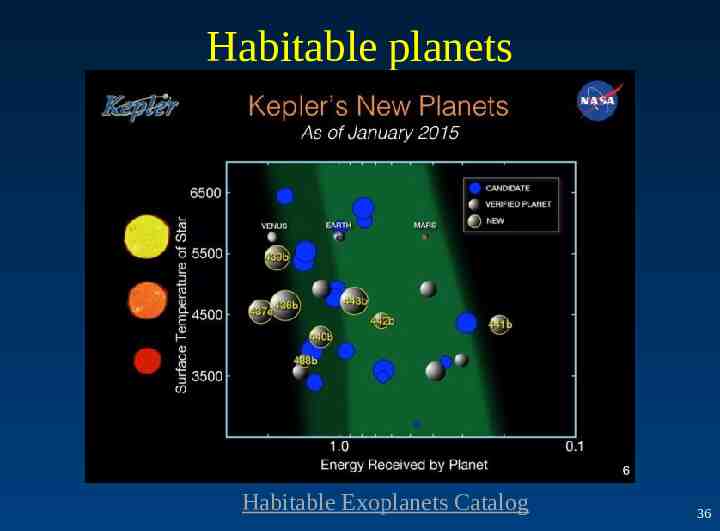
Habitable planets Habitable Exoplanets Catalog 36
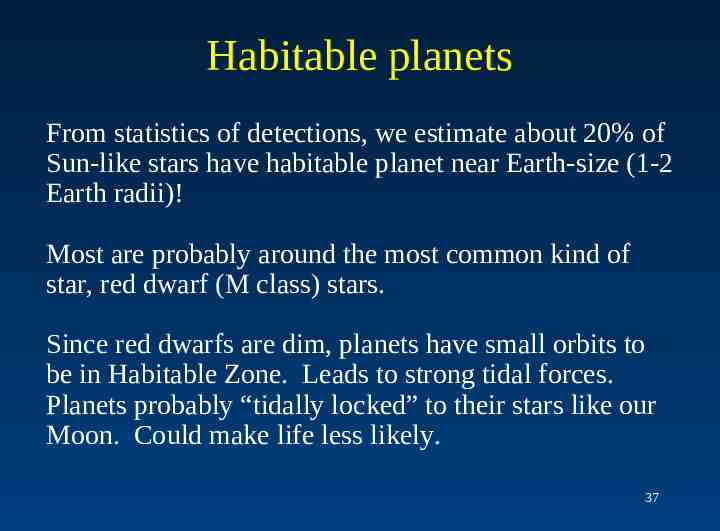
Habitable planets From statistics of detections, we estimate about 20% of Sun-like stars have habitable planet near Earth-size (1-2 Earth radii)! Most are probably around the most common kind of star, red dwarf (M class) stars. Since red dwarfs are dim, planets have small orbits to be in Habitable Zone. Leads to strong tidal forces. Planets probably “tidally locked” to their stars like our Moon. Could make life less likely. 37
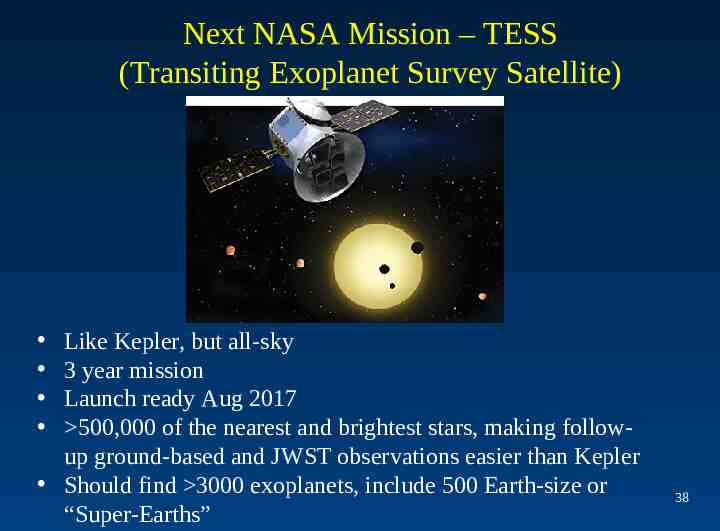
Next NASA Mission – TESS (Transiting Exoplanet Survey Satellite) Like Kepler, but all-sky 3 year mission Launch ready Aug 2017 500,000 of the nearest and brightest stars, making followup ground-based and JWST observations easier than Kepler Should find 3000 exoplanets, include 500 Earth-size or “Super-Earths” 38
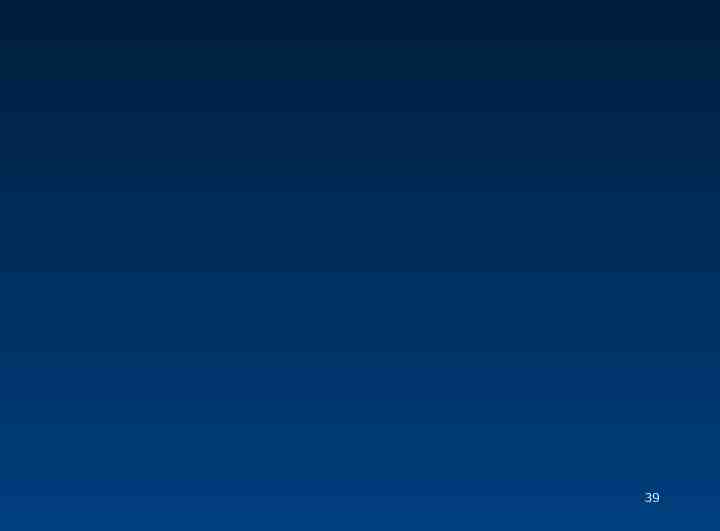
39
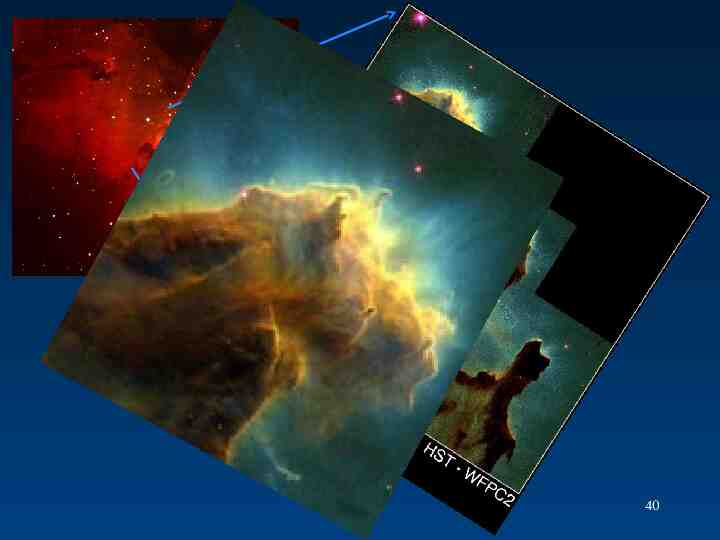
40
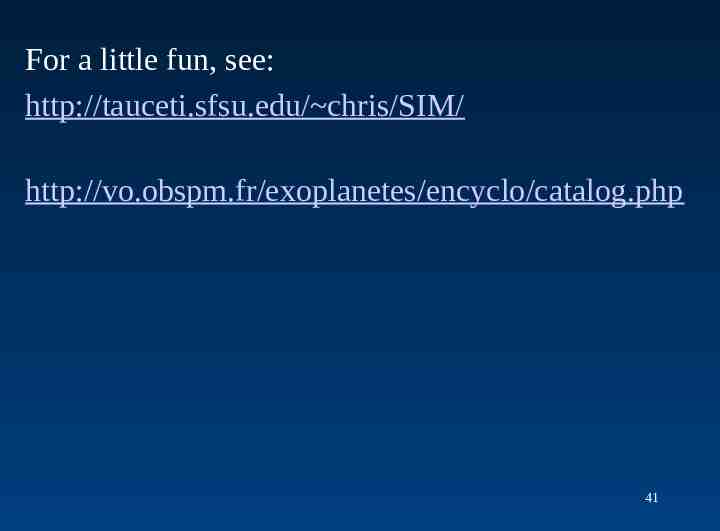
For a little fun, see: http://tauceti.sfsu.edu/ chris/SIM/ http://vo.obspm.fr/exoplanetes/encyclo/catalog.php 41
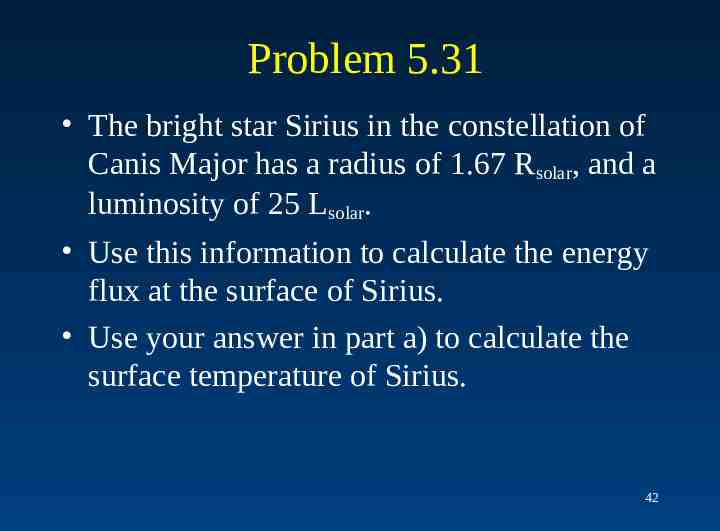
Problem 5.31 The bright star Sirius in the constellation of Canis Major has a radius of 1.67 Rsolar, and a luminosity of 25 Lsolar. Use this information to calculate the energy flux at the surface of Sirius. Use your answer in part a) to calculate the surface temperature of Sirius. 42
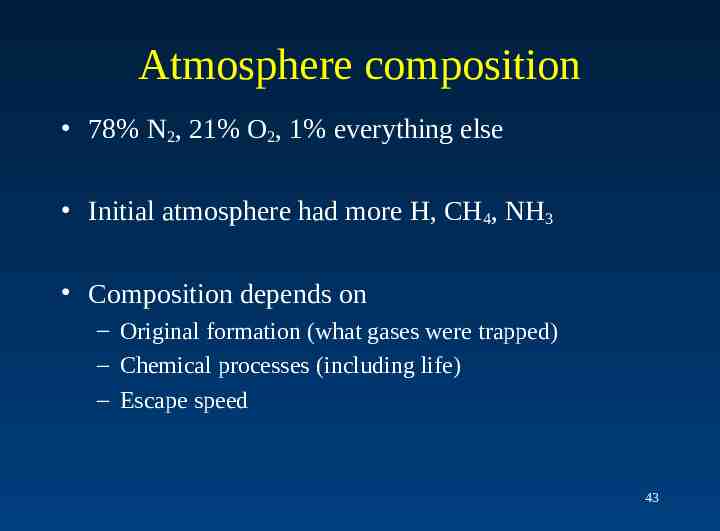
Atmosphere composition 78% N2, 21% O2, 1% everything else Initial atmosphere had more H, CH4, NH3 Composition depends on – Original formation (what gases were trapped) – Chemical processes (including life) – Escape speed 43
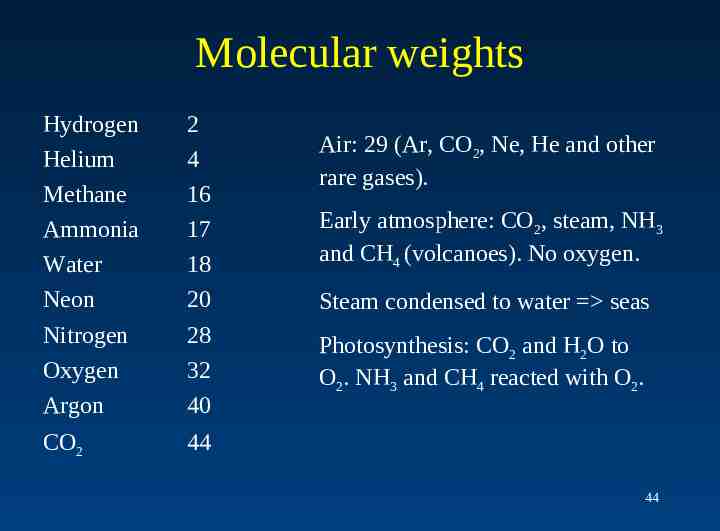
Molecular weights Hydrogen Helium Methane Ammonia Water Neon Nitrogen Oxygen Argon 2 4 16 17 18 20 28 32 40 CO2 44 Air: 29 (Ar, CO2, Ne, He and other rare gases). Early atmosphere: CO2, steam, NH3 and CH4 (volcanoes). No oxygen. Steam condensed to water seas Photosynthesis: CO2 and H2O to O2. NH3 and CH4 reacted with O2. 44
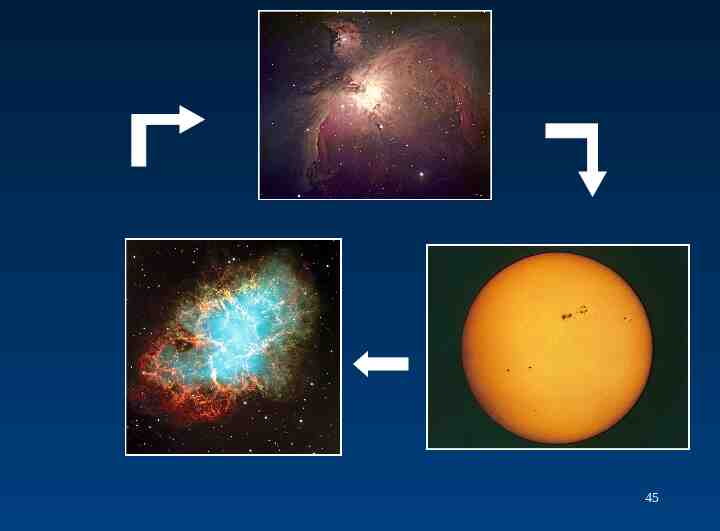
45
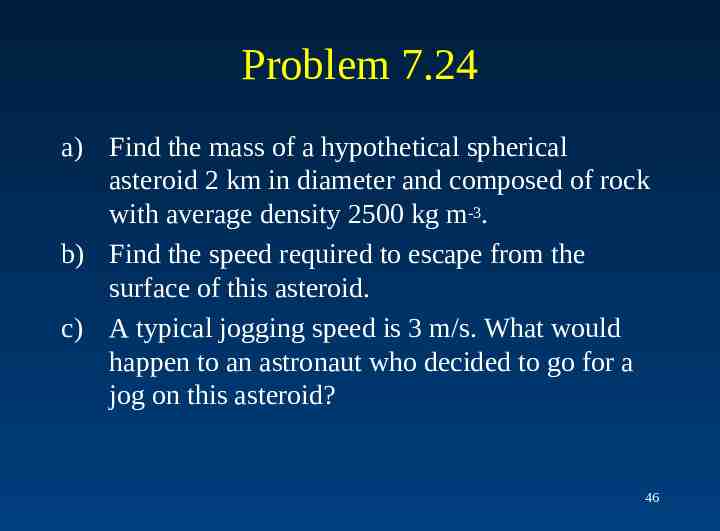
Problem 7.24 a) Find the mass of a hypothetical spherical asteroid 2 km in diameter and composed of rock with average density 2500 kg m-3. b) Find the speed required to escape from the surface of this asteroid. c) A typical jogging speed is 3 m/s. What would happen to an astronaut who decided to go for a jog on this asteroid? 46
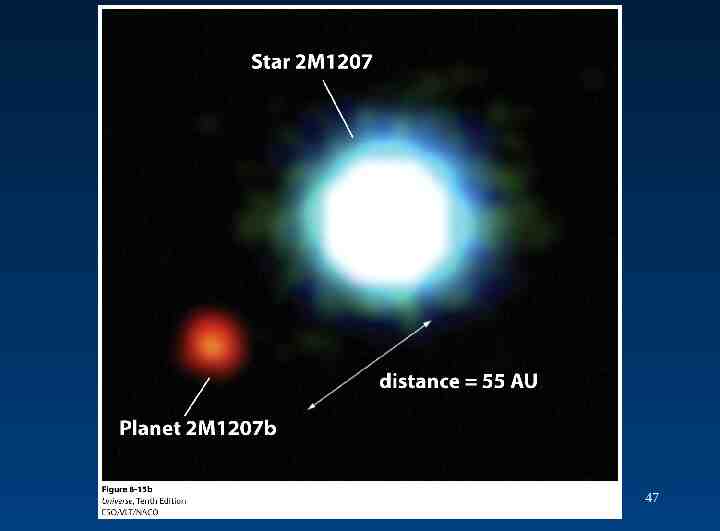
47